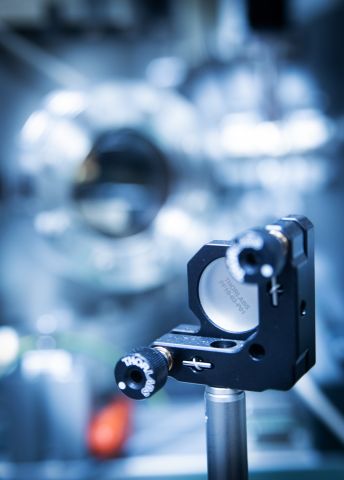
Project Grant 2022
Entanglement and decoherence in ultrafast electron spectroscopy and microscopy
Principal investigator:
Mathieu Gisselbrecht, Associate Professor
Co-investigators:
Lund University
Cord Arnold
Anne L'Huillier
Anders Mikkelsen
Claudio Versozzi
Institution:
Lund University
Grant in SEK:
SEK 26.1 million over five years
Quantum mechanics – the branch of physics that describes how particles like electrons and atoms behave – has had a huge impact on society since its inception in the first half of the 20th century. Lasers and transistors – the building blocks of modern electronics – are examples of inventions that have become a reality thanks to an understanding of matter at the quantum level.
Intensive research is currently in progress to use quantum properties of individual particle systems to construct e.g. ultrafast quantum computers, eavesdropping-proof quantum encrypted communication, and sensitive measuring methods.
Many of these applications are based on quantum entanglement. Simply put, entangled particles behave as though they were connected together, thus impacting each other even when far apart.
“Entanglement is one of the most fascinating properties of the quantum world, but it is not entirely understood. We are developing new methods to study entanglement in matter and how it disappears,” says Mathieu Gisselbrecht, who is heading the project at Lund University.
Entangled states are very fragile, and are easily and quickly destroyed by external factors, such as e.g. when particles interact with other particles at room temperature.
To follow these rapid changes of entanglement, the researchers are using ultrafast light pulses, known as attosecond pulses, which last for only a billionth of a billionth of a second. These short light pulses enable them to create entangled quantum systems by ionizing matter, i.e. by emitting one or more electrons. They then study how entanglement occurs and disappears at room temperature using, among the several possible available methods, primarily electron spectroscopy.
Tailored light pulses
Electron spectroscopy is a well-established analytical method based on the photoelectric effect, whereby light shining on a material knocks out electrons from the sample. By determining the energy required to emit the electrons, electron spectroscopy can provide information about which atoms make up the material and on the material’s optical and magnetic properties.
The researchers at Lund are now developing a method that will enable them to obtain even more information than before from electron spectroscopy.
“We are using tailored sequences of attosecond pulses with laser light at different wavelengths to measure not only the energy of the emitted electron but also to characterize its quantum state. We recently demonstrated that by combining attosecond research and electron spectroscopy in this way, we can obtain quantum information in small quantum systems. This is what we are going to look into further,” Gisselbrecht says.
Using electron spectroscopy, the researchers in the project are also developing ways to measure the evolution in time of coherence, i.e. electrons that oscillate with a constant phase difference, this may yield additional knowledge about the dynamics of entanglement.” Gisselbrecht elaborates:
“In this way we can get information even about ultrafast processes that may occur in the transient regime, and before our final measurement of the system’s quantum state at long times.”
The project includes researchers concentrating on developing the light source – the laser – so it measures specific types of atoms with X-rays.
Studying semiconductors
Initial experiments in the project will focus on small systems – atoms and small molecules. The researchers will then address more complex situations, such as semiconductors in nanometer structures.
To understand the behavior of entanglement in these structures, the team has among its members researchers with expertise in developing attosecond-pulses for electron microscopy.
“Microscopy is crucial, so we can see the surroundings, and not just record a signal that we do not know where it originates from. For example, microscopy enables us to see which part of a nanowire coherently emits an electron, or whether loss of coherence occurs only on one side of the nanowire.”
The project also contemplates the development of theoretical approaches for a description of entanglement. Theoretical researchers in the project are developing models that can predict the situations in which entanglement could occur, e.g. which light sources and sequences of light pulses are suitable to create entangled states. The experimental researchers will be able to use that information as guidance and try to confirm it in their experiments.
Paving the way for others
In the long term, this research may contribute to the development of new materials in which entanglement is used for new applications, such as quantum computers. But it may also be put to more immediate use, as Gisselbrecht explains:
“Our work is paving the way for use of light sources to create and study entanglement. These methods can be used by us and others, and will hopefully contribute to a better understanding of fundamental properties of different phenomena and different types of materials, for example superconductivity, which is often used today in the development of quantum computers.”
Text Sara Nilsson
Translation Maxwell Arding
Photo Åsa Wallin