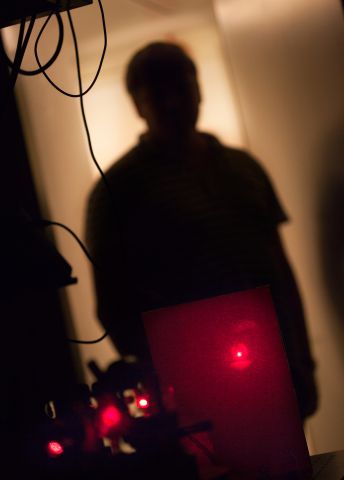
Project Grant 2014
Anisotropic forces in colloid chemistry
Principal investigator:
Peter Schurtenberger, Professor of physical chemistry
Co-investigators:
Johan Bergenholtz
Jerome Crassous
Per Linse
Tommy Nylander
Ulf Olsson
Emma Sparr
Anna Stradner
Håkan Wennerström
Institution:
Lund University
Grant in SEK:
SEK 42.4 million over five years
Colloids are small particles, from a few nanometers to around a micrometer in diameter. They move freely in a gas or liquid without forming clumps or sinking, and they are found in many places in the natural environment and in industry. Some examples are milk, where proteins form colloids, and paints, where the pigment is in the form of colloids dispersed throughout the solvent.
So colloids are of great practical importance. But for many years, researchers have also used them as a model for atoms and proteins. The movements of colloids, and how they affect each other, have been studied, and conclusions have been drawn as to how small particles behave, for instance in crystals.
“Many important materials crystallize when they change from liquid to solid form. Steel and water are two examples. It is of great interest to know how this process works, but extremely difficult to visualize atoms and molecules. Colloids, on the other hand, can be seen under a microscope. If they are good models for the atom in question, you can observe the colloids instead – the way they move during crystallization,” explains Peter Schurtenberger, Professor of Physical Chemistry at Lund University.
A model that does not always work
Are colloids good models then? Yes and no. They have taught researchers quite a bit about materials such as window glass, which is a solid, but shares certain properties with liquids. But for many processes colloids are not nearly good enough. Colloids are generally spherical, shaped like small balls. But molecules are not round like balls; their ends may be shaped in a number of ways. Moreover, both molecules and proteins may have electrical charges unevenly distributed in them, and have binding sites that are important when they interact with the world around them. Colloids reflect none of this. This is what Peter and his colleagues want to change.
Irregularity produces different properties
In a research project funded by the Knut and Alice Wallenberg Foundation, they will be analyzing the properties of atoms, molecules and proteins that influence their interaction with their surroundings. Anisotropy is the term used when a material has different physical properties in different directions, and this is the subject into which the researchers in Lund will be delving.
“What effect does it have when we move from something that looks like a billiard ball to something that has ‘sticky patches’ on it? How does this affect a protein’s movements in a cell, for example, or how particles pass through a cell membrane?”
Transport over cell membranes is central to many of the body’s processes, and determines how drugs work, for example. At present, the molecules that carry drugs are often spherical, but anisotropy, i.e. irregular shapes, might enable the drug to penetrate more effectively. The researchers in Lund will be experimenting with artificial colloids to find out how they behave when they meet a cell membrane. In this way the team aims to ascertain whether it would be better to design drug particles as ellipsoids, for instance, or like red blood cells: round, flat and thicker around the edges than in the middle.
Tailored colloids at the forefront of research
First of all, the researchers build model systems, both in reality and digitally, so as to understand which properties are important.
“Look at the molecules that form the capsule of a virus. They are wonderful – well-designed, complex, and they put themselves together! We researchers cannot get a material to make itself simply by pouring things into a test tube. In this project we want to understand what is needed at atomic and molecular level so that a material will behave in this way,” Peter explains.
The next stage will be for them to try to design colloids that really look like atoms and molecules. It is possible in the laboratory to modify the size of colloids, to make them react to temperature or salinity, grow and shrink, collapse and swell. It is possible to manufacture nanoscale magnetic colloids, whose direction is affected by that of other particles, just as when charged proteins and molecules meet.
The project brings together many fields of knowledge: biophysical chemists, who are working on proteins and membranes, colloid experts and theoreticians, who are skilled at simulation. The research is divided into a number of sub-projects, Peter stresses that no single research team would have been able to carry out any of them.
“All the projects are right on the limits of what is possible at present. They really all are at the cutting edge of research. I firmly believe that the new knowledge, and the complex colloids we are creating, will lay the foundations for more interesting and valuable materials in the future.”
Text Lisa Kirsebom
Translation Maxwell Arding
Photo Magnus Bergström