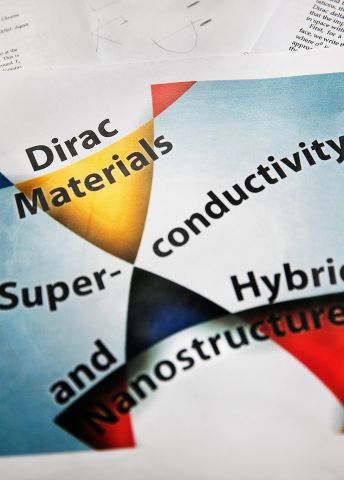
Project Grant 2013
Functional Dirac Materials
Principal investigator:
Alexander Balatsky, Professor of Theoretical Physics
Co-investigators:
KTH Royal Institute of Technology
Oscar Tjernberg
Johan Åkerman
Uppsala University
Annica Black-Schaffer
Chalmers University of Technology
Floriana Lombardi
Institution:
KTH Royal Institute of Technology
Grant in SEK:
SEK 32 million for a five-year project
Long handwritten equations fill the whiteboard in Alexander’s office at the AlbaNova University Center. In the room are also a number of computer screens that he uses as an aid in his theoretical studies of how materials behave at the atomic level.
“Here you can see what we are doing,” Alexander says, and runs a film in which myriad red and white dots move through a blue lattice. The film, which took a couple of months to make, is based on advanced calculations made on a supercomputer.
“What we see is a membrane made of graphene, which is a Dirac material. Here we simulate the water that can flow through the nanopores of the material. We think that one future application is desalination or removal of arsenic from drinking water. DNA sequencing is another possibility.”
Properties with great potential
Dirac materials are a class of complex and functional nanomaterials offering great potential in the development of new electronic components. These materials have properties not found in current electronic materials. The properties occur thanks to strong links between spin, charge and structure in the materials, which creates the potential for developing magnetic or superconductive composites, Alexander explains.
“All the electronic equipment in our lives exists because we have learnt how to handle modern materials. We can manufacture them and give them specific functions. It is that ability – to be able to manipulate materials – that has led to the enormous technological developments we have seen over the past 100 years.”
Alexander is Professor of Theoretical Physics at KTH Royal Institute of Technology, and Nordita, the Nordic Institute for Theoretical Physics. His research field is condensed matter physics, which involves the study of materials in solid and liquid state. In the 2000s he began to see a pattern whereby different nanomaterials exhibited the same characteristics. He later gave the group the name Dirac materials after the founder of quantum mechanics, Paul Dirac, who was awarded the Nobel Prize in Physics in 1933.
Among other things, Dirac derived the Dirac Equation, a relativistic equation for calculating the wave function of electrons, and a cornerstone of quantum mechanics. The equation can also be used to model materials.
Seeking robust performance
The Dirac Materials research project has been awarded funding over five years by the Knut and Alice Wallenberg Foundation.
“We have put together a really good team for this project, with two theoreticians and two experimentalists. The grant enables us to adopt a more pro-active approach to understanding and testing Dirac materials, using this as a basis for designing new materials and nanostructures that derive benefit from Dirac properties,” Alexander adds.
The team is studying how different properties affect the material’s performance, which is important for future applications.
“For instance, if you add more magnetism, you want a robust response from the material in order to achieve an enhanced function. Only then will you be able to use it in new products and applications.”
Dirac materials include graphene, topological insulators and certain unconventional superconductors (see fact box). These are currently among the most studied materials in the field of condensed matter physics.
A common feature of Dirac materials is that they have few points (‘Dirac points’) where changes affect the response of the material. The materials are therefore particularly useful if the aim is to change and control properties. When using conventional metals it is usually necessary to deal with numerous points in the interstices and surfaces of the materials.
“There is a surface called the Fermi surface, which characterizes the behavior of a metal. In Dirac materials this surface has been shrunk to a single point. We can control these sensitive points using the math in the Dirac equation,” Alexander explains, and draws the points on the board.
Rapidly growing research field
The project involves basic theoretical and experimental materials research. The team hopes that the research will also yield some specific components with useful applications such as spintronics, electronics and desalination using nanopores.
“The goal is to study unconventional superconductors on a nano-scale, and see exactly what controls stability at the Dirac points. Another angle is to examine spintronics in topological insulators and see what potential they offer. We also want to study crystalline topological insulators, a new material.”
Alexander mentions that one major challenge in researching into Dirac materials is keeping abreast of developments in the field. Rapid changes are occurring, not least in the use of grapheme, and it is difficult to say which route forward offers the greatest potential.
“Yet it is important that we reach a phase where we can either show some new phenomena or new applications for graphene and topological insulators. That would be truly great.”
Text Susanne Rosén
Translation Maxwell Arding
Photo Magnus Bergström
Facts:
Nanomaterials are structures having very small dimensions, between 1 and 100 nm (1 nm = a millionth of a meter, roughly one-thousandth of a human hair).
Graphene is a two-dimensional nanomaterial that is only one layer of atoms thick, is very strong, and has excellent conductivity.
Topological insulators have the unique property that they cannot conduct current inside themselves, only on their surface. One example of unconventional superconductors is d-wave superconductors, a form of high-temperature superconductor where the electrons travel in a circuit resembling a four-leaf clover.
Spintronics (or magnetoelectronics) combines microelectronics, which is based on the charge of the electronics, with the magnetism that is created by the electrons’ own spin (rotation).