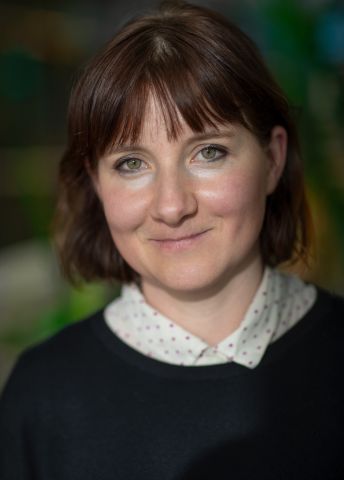
Joanna Rorbach
Associate Professor of Molecular Biology
Wallenberg Academy Fellow/Wallenberg Scholar
Institution:
Karolinska Institutet
Research field:
Molecular metabolism
Wallenberg Academy Fellow/Wallenberg Scholar
Institution:
Karolinska Institutet
Research field:
Molecular metabolism
Rorbach opens the lid of a cell culture dish. She attaches a tip to the pipette and uses it to draw up pink culture fluid.
Here, in the lab at Karolinska Institutet’s research building Biomedicum, her research team is culturing cells. From the cells, they then extract the structures on which their research focuses: mitochondria.
Mitochondria are the cell’s power producers. They convert nutrients from the food we eat, and oxygen from the air we breathe, into an energy form that the body’s cells can use.
Mitochondria also contain their own genome, DNA. The mitochondrial DNA carries information to make key proteins needed for cellular energy production.
Rorbach is studying how proteins are made in human mitochondria. This process differs from “normal” protein production in the cell, which takes place outside the mitochondria, and is a complex mechanism.
“The mitochondrial genome produces only thirteen proteins. But to manufacture them, hundreds of proteins from outside the mitochondrion must be imported at the right moments. We’re beginning to understand this process in more detail, but there’s still much we don’t know. It’s a really exciting new area.”
If the mitochondria do not work appropriately, symptoms may result almost anywhere in the body. Energy-intensive functions, like the muscular contraction and neuronal function, are particularly affected. In recent years, ageing and cancer have also been linked to mitochondrial dysfunction.
“People used to wonder why I spent so much time on mitochondria, but now it’s easier to explain that these are really important processes. Thankfully wow we also have new techniques for addressing questions that were previously difficult to answer.”
Amongst other things, Rorbach is examining the structures that translate genetic information into proteins; the mitochondrial ribosomes. Ribosomes are made up of large numbers of proteins and other building blocks. A new imaging technology, cryo-electron microscopy (cryo-EM), provides detailed information about their atomic structure.
“With this technique, we can visualize the assembly of ribosomes, and see exactly where and how a given protein binds. Previously, it was a question of guesswork based on indirect clues. We started using this technique recently and it is fascinating to see now how different the mitochondrial protein synthesis machinery really is.”
Human mitochondria are thought to have originated from bacteria taken up by primitive cells millions of years ago. They have evolved considerably since then and using cryo-EM the researchers can elucidate the unique features of human mitochondrial protein synthesis.
Cryo-EM is also the reason that Joanna Rorbach is working in Stockholm. She was born and raised in Poland but received her PhD and worked as a postdoc in the United Kingdom, in Newcastle and Cambridge.
Towards the end of her postdoc period, biological researchers were beginning to use cryo-EM to study mitochondrial ribosomes. Rorbach was keen to learn more and visited SciLifeLab in Stockholm – a new center for cryo-EM. The idea then was that she would return to Cambridge, but when she was offered a position at Karolinska Institutet, she jumped at the chance.
“I knew without doubt that moving here would be a good idea. I saw that the environment offered great potential, with all its facilities and collaborating groups.”
Other techniques that have changed the team’s approaches are powerful new methods for sequencing DNA, and its working copy, RNA. Now, if something goes wrong during mitochondrial protein synthesis, sequencing techniques can be used to find the precise point along an RNA strand where the ribosome stops.
The team is also using the new genome editing technique Crispr/Cas9. This makes it easy to cut a specific gene out of the genome, allowing researchers to see what happens when the cell no longer synthesizes the protein corresponding to that gene.
“Crispr has many advantages over the systems we used before. Now we can efficiently and rapidly knock out proteins of interest to understand their functions.”
“The grant provides me the security and freedom to pursue important questions in human health. It will allow me to expand my research team and develop new, unexpected lines of research that appear along the way.”
Rorbach’s research may also provide clues for the generation of new drugs.
It is now known, for example, that mitochondrial protein synthesis – translation – is increased in different types of cancer. Here, knowledge of the molecular structure of the machinery involved may be useful.
“It improves our chances of designing molecules that can stop protein synthesis and serve as treatment. When we have a more thorough understanding of the machinery, we will also be able to contribute to development of drugs able to restore protein synthesis in patients with impaired mitochondrial function.”
Rorbach enjoys spending her afternoons in the lab and is happy to continue doing so.
“I love being in the lab – that’s where the fun stuff happens.”
Text Sara Nilsson
Translation Maxwell Arding
Photo Magnus Bergström
Instructions for the design of our proteins – which amino acids will be used to build them – are found in our DNA. When proteins are built, a working copy of the DNA is created, called mRNA.
In the protein factory – the ribosome – the information in the mRNA molecule is then translated into the correct sequence of amino acids, ultimately resulting in a protein.
The process by which ribosomes use mRNA to build proteins is called translation.