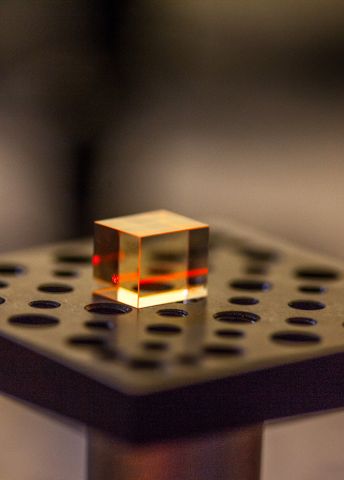
Project Grant 2016
A new generation of slow light systems
Principal investigator:
Stefan Kröll, Professor of Atomic Physics
Co-investigators:
Lund University
Lars Edvinsson
Mats Gustafsson
Solveig Melin
Tyndall National Institute and University College Cork
Stefan Andersson-Engels
Caltech
Lihong Wang
Ecole Nationale Supérieure de Chimie de Paris
Philippe Goldner
Observatoire de Paris
Yann Le Coq
Institution:
Lund University
Grant in SEK:
SEK 36.4 million over five years
The Lund researchers have spent several years working on new materials at the atomic level. They wanted to create a type of crystal by precisely changing the energy of certain atoms in the material, so that it allows light to pass through at a certain frequency. When they succeeded, something surprising happened.
“It turned out by chance that the light penetrating the crystal also slowed down a lot. In materials like glass or water light slows down to just over half-speed. But in our material the speed was only one ten-thousandth,” explains Stefan Kröll.
He is a professor at the Department of Atomic Physics at Lund University, and is now co-coordinating a team of researchers from Lund with fellow researchers in California and Paris. With the help of funding from the Knut and Alice Wallenberg Foundation, they will be examining how slow light can be used.
New image and treatment potential
One potential application is in medical imaging technology. Many research teams would like to be able to scan the body using laser, and analyze the laser light that emerges to obtain images of changes in organs, for example.
The problem is that the light scatters in tissue and changes direction several times. It is therefore necessary to identify the light that has actually penetrated the part of the body being examined.
One solution is to also send an ultrasound pulse toward the area to be examined. The frequency of the light shifts very slightly when the paths of the light and the sound cross. This tells us which part of the exiting light has passed through the target tissue; that light will have a new frequency. But in practice this is complicated, because the frequency-shifted light is only a tiny proportion of all the light, and is difficult to distinguish. This is where the material developed by the Lund team comes into the picture.
“Our crystals act as a filter that lets the shifted frequency through, and strongly suppresses other light. But a lot of unshifted light still makes it through. That’s where the slower light speed is a help. We have designed the crystal for the shifted light, so that is the only light that’s slowed down, which means it comes out long after the unshifted light,” Kröll says.
According to the team’s calculations, the method they are working on ought to produce much better images from inside the body than those obtained at present.
“We’re also trying to find a way to focus the light more using the crystals. It would be better if it traveled in a straight beam instead of ricocheting around in the tissue. But we don’t know yet whether it will work.”
One use of this kind of technology would be to treat tumors deep inside the body using laser.
Measuring time using frequencies
A quite different application for slow light is to measure time. We need to measure time very precisely so that GPS and other navigation systems will work, and to ensure rapid data communication, for example.
In practice, time is measured by measuring frequencies in various ways. Old-fashioned grandfather clocks were based on the frequency of the pendulum. Modern-day measurement of time is based on atoms, which emit light at a very precise frequency.
To be sure that the system provides the right information, it is necessary to know if the frequency changes. But a reading taken in the atomic system itself causes disruption. To avoid having to take readings more often than needed, a kind of monitoring station is built. This is a cavity through which light from the atoms is channeled. The cavity is made to a length corresponding exactly to a predetermined number of oscillations of the light, which means that a standing wave is created. If the frequency changes, there will be no standing wave, which signals it is time to take a reading in the system.
Potential for more stable systems
Unfortunately, there is another way the standing wave can disappear, namely if the length of the cavity itself changes. Since the changes involved are infinitesimally small, less than the radius of an atomic nucleus, it is difficult in practice to maintain a sufficiently stable cavity. Minute changes in temperature or air pressure suffice to destabilize a cavity, so it is kept in a vacuum.
This is where another property of the new material developed in Lund could be of benefit. If light is allowed to pass through one of the crystals instead of a cavity, the sensitivity to changes in length decreases by about ten thousand times. This means that the margins are ten thousand times greater. This might create opportunities for a completely new and more stable system for measuring time.
“There are bound to be many challenges ahead that we’re not even aware of yet. But there is an enormous potential, so it’s obviously worth investigating. The idea of getting light to take a short route through tissue, for instance – It’s a pretty wild notion. But we want to take a look at it anyway,” Kröll explains.
Text Lisa Kirsebom
Translation Maxwell Arding
Photo Magnus Bergström